One of the well-known reactions in C-C bond formation is aldol reaction. This robust reaction has a great potential in controlling two stereocentres of the product.
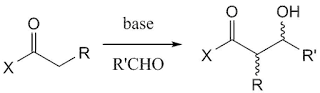 |
General aldol reaction |
The control on the stereocentres of the product is determined from the stereochemistry of the formed enolate;
Z- and
E- enolates would give different control on the outcome of aldol products.
In the enolate formation, there are two possible forms of possible enolate; it could be
E- or
Z- enolate. Therefore, it is important to be able to control enolate geometry. The control of enolate geometry can be done by changing the reaction conditions such as solvent.
 |
Enolate formation in different solvents |
The example above shows the enolate from methyl propanoate in THF favours
E-enolate, but in solvent mixture of HMPA/THF
Z-enolate is favoured. This control on enolate geometry can be rationalised by looking into its mechanism.
 |
The general mechanism of enolate formation (top) and the stereoelectronic requirement in enolate formation (bottom) |
Under this condition, the deprotonation proceeds via a cyclic transition state and to accomodate the deprotonation the acidic proton needs to be line π(C=O) orbital. From the mechanism above, there are two possibilities of the 6-membered chair type transition state where R eclipsing X or 1,3-diaxial interaction between R and
iPr group.
 |
The 6-membered chair type transition in enolate formation |
From those transition states, it can be rationalised that in THF it proceeds via tight-ion coordination which makes a severe 1,3 diaxial interaction; 1,2 interaction is not as bad as 1,3 diaxial interaction. Hence,
E-enolate is favoured in THF. Meanwhile in THF/HMPA,
Z-alkene is favoured because HMPA, which is a good Lewis base, coordinates to Li ion reducing the coordination to NR
2; hence the transition state is opened up. This means 1,3 diaxial interaction is less severe; hence,
Z-enolate is favoured. Furthermore, using larger bases would favour
E-enolate while larger X would favour
Z-enolate.
 |
Enolate formation in different bases and R groups.
Reference: C. H. Heathcock, C. T. Buse, W. A. Kleschick, M. C. Pirrung, J. E. Sohn, and J. Lampe, J. Org. Chem., 1980, 45, 1066-1081. |
After understanding how to control the geometry enolate, it is time to bring the second piece of aldol reaction which is the addition to the aldehyde compound. As mentioned earlier, the stereochemistry of the aldol product depends heavily on the geometry of enolate. In aldol reaction,
Z-enolate would lead to
syn-aldol while
E-enolate would lead to
anti-aldol.
 |
Different relative stereochemistry of aldol products |
It is important to note that the stereochemistry of aldol product is the relative stereochemistry which means its enantiomer is also formed.
To rationalise the stereochemistry of aldol product, it is important to see the aldol reaction in detail. Firstly, in aldol reaction metal ion coordinates to both reacting partners. This coordination brings both of the reacting partners in close proximity. Then, a concerted electrocyclic reaction happens to form the aldolate product.
 |
Mechanism of aldol product formation |
In the second step, the conformational energies of the 6-membered transition state dictate the selectivity the aldol reaction.
In
Z-enolate there are two possible orientation of the aldehyde to be added by the enolate.
 |
The 6-membered chair type transition state of aldol reaction with Z-enolate |
As mentioned before, the conformation energies of the transition state dictate the selectivity of the reaction. In this case, the transition state
3 has higher conformation energy than
1 due to severe 1,3-diaxial interaction between R and R'' groups. This means the formation of
2 (
syn-aldol) is favoured rather than
4 (
anti-aldol). The same principle is applicable to rationalise the stereochemistry outcome from
E-enolate.
 |
The 6-membered chair type transition state of aldol reaction with E-enolate |
The extent of diastereocontrol is controlled by 1,3-diaxial interaction where larger group gives better diastereocontrol as shown below.
 |
Aldol reaction with bulky R group |
Additionally, the enantiomer of both products are formed and this is due to the transition states are also enantiomer which means they have the same energy.
 |
Enantiomeric relationship of the transition state leads to racemic mixture |
However, this symmetry can be broken by using a chiral aldehyde or chiral enolate. The use of chiral aldehyde or chiral enolate makes the transition states are no longer enantiomeric but diastereomeric which means they have different energies. Furthermore, the aldol reactions with chiral aldehyde are assumed under Felkin-Anh (FA) control.
 |
Aldol reaction using chiral aldehyde |
Furthermore, by using chiral enolate it introduces more control at the chiral centre of enolate or carbonyl.
 |
Aldol reaction using chiral enolate |
The example above shows the chiral centre with bulky
tBu group makes
Z-enolate favourable and it also directs the attack from front side by providing steric hindrance to disallow backside attack.
Comments