In the late 20th century, Jean-Marie Lehn coined the definition of supramolecular chemistry as "
the chemistry of molecular assemblies and of the intermolecular bond". In this new type of "molecules" the main stabilisation factor is not solely on the covalent chemistry but the intermolecular interactions such as hydrogen bonding and van der Waals interactions. As a side note, Jean-Marie Lehn was awarded the 1987 Nobel Prize in Chemistry with Donald J. Cram and Charles J. Pedersen for "
their development and use of molecule with structure-specific interactions of high selectivity".
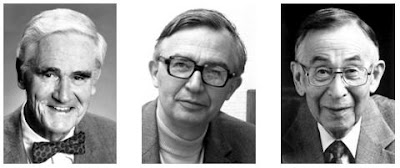 |
Donald J. Cram, Jean-Marie Lehn and Charles J. Pedersen (left to right) |
One of the examples of supramolecular chemistry is self-assembled metal cage complex which has been a great interest recently.
 |
Examples of metal cage complexes |
Metal cage complexes have been great interest in the last decade due to vast amount possible geometries that can be explored. This vast geometries is contributed from the fact that transition metal centre can afford higher number coordination number relative to organic-based self-assembled complexes. High coordination number of metal centre implies that the metal cage complexes are not limited to 2D but it can form 3D structures such as tetrahedron and cubic. The driving force of the formation of metal cage complex is the intermolecular interactions, as mentioned before, such as π-π interactions that arises from the intertwined ligands.
One of the important factors in metal cage complex synthesis is designing the appropriate ligand. A careful design on the ligand is necessary to ensure the formation of metal cage complex. As shown in
2, the general design on the ligand for metal cage complex is two bidentate ligands connected by rigid aromatic spacer.
 |
The ligand for metal cage complex 2. The bidentate ligands are highlighted in red and the aromatic spacer in blue. |
The used of bidentate ligand in the metal cage complex is due to stronger interaction, that arises from chelating effect, with metal centre. This strong interaction between metal and ligand is one of the stabilisation factor of the metal cage complex; this makes the metal cage complex is the most thermodynamically stable product in the synthesis. This means choosing the correct metal centre is affecting the options for the ligand. A labile metal centre is needed which gives the complex the opportunity to rearrange to form the most thermodynamically stable product.
Another design feature in the ligand is the rigid aromatic spacer that connecting the ligands. The rigidity of the ligand is important in metal cage formation as it disallows both chelating agents to bind with the same metal centre. This effect can be observed in enterobactin analogue complex
5 and
7 below.
 |
The synthesis of enterobactin analogue 5 and tetrahedron cage 7. |
The removal of CH
2 group from the linker would give
7 a more rigid structure and this rigid structure would disallow three catechol units to bind with the same metal centre. Hence, it is important to get the right flexibility of the ligand to ensure the metal cage complex is formed. Moreover, the extended aromatic spacer enhances the stability of the metal cage complex due to π-π interactions between those aromatic spacers.
Another interesting area of self-assembled metal cage complex is its synthesis. A Careful design of the reaction conditions, especially the stoichiometry of the metal centre and the ligand, is needed to ensure the formation of the metal cage complex. The synthesis of metal cage complex is thermodynamically-driven reaction which means the metal cage complex needs to be the most thermodynamically stable product. This means, as mentioned earlier, labile metal centre is preferred as it gives the opportunity of the complex to rearrange forming the most stable product.
There are two general approaches to synthesise metal cage complex. First approach is direct synthesis where metal centre and the ligand is reacted; this method is commonly used in metal cage complex synthesis. In this synthesis, the ligand needs to be synthesised first then the formed ligand is reacted with the metal centre, with the appropriate stoichiometry, to give the metal cage complex product. The example of this approach is the synthesis of
2, where ligand
3 is synthesised first (6 steps) then reacted with Co(II) ions.
 |
Synthesis of cubic metal cage complex 2. |
The second approach forming metal cage complex is using sub-components of the ligand and the metal centre. In this method, the synthesis of the ligands and the cage complex happen in one-step reaction.
 |
Synthesis of tetrahedron cage 11. |
In the reaction scheme above, there is no apparent chelating agent within the reagent of the synthesis of
11. The imine formation between
9 and
10 gives pyridine-imine as the chelating agent as well as the connecting group to form metal cage complex. Besides that, the alcohol side-chain acts as solvating group of the complex as well as providing the hydrophobic environment within the cage. The formation of the tetrahedron complex might also be driven by template effect of tetrahedron sulphate anion in this reaction.
The self-assembled metal cage complex is still its infancy and there are many areas that can be explored. The hydrophobic cavity provides interest to use the metal cage complex as host for small molecules such as phosphate esters. The fact that it can act as host for small molecules, metal cage complex can be used as catalyst where the metal cage tightly bind the substrates and fast release of the product which makes it another option to mimic enzyme catalysis. Moreover,
2 has been showed to catalyse Kemp elimination reaction by factor 10
5.
 |
Kemp elimination catalysis by 2 |
References
- M. Whitehead, S. Turega, A. Stephenson, C. A. Hunter and M. D. Ward, Chem. Sci., 2013, 4, 2744-2751.
- D. L. Caulder, C. Brückner, R. E. Powers, S. König, T. N. Parac, J. A. Leary and K. N. Raymond, J. Am. Chem. Soc., 2001, 123, 8923-8938.
- J. L. Bolliger, A. M. Belenguer and J. R. Nitshcke, Angew. Chem., Int. Ed., 2013, 4, 2744-2751.
- W. Cullen, M. C. Misuraca, C. A. Hunter, N. H. Williams and M. D. Ward, Nat. Chem., 2016, 8, 231-236.
Comments