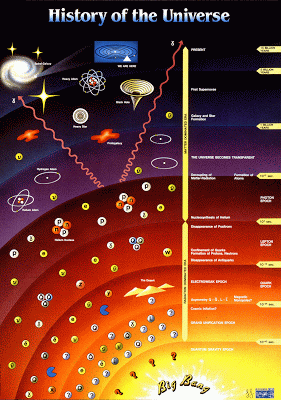 |
History of the universe |
In this section, we will try to see the trends in atomic properties of the periodic table, especially on
s and
p-block elements. The properties that wee will have a look are radius, ionisation energy, electron affinity, and electronegativity. Besides that, we will see how the electronegativity can predict the element characterisitic (metal, metalloid, and non-metal). Before we see its trends, we will have a quick journey at beginning of the universe.
At the beginning of the universe, there were no elements or atomic nuclei; the atomic nuclei were formed around 1 minute after the big bang. Around 1 minute after the big bang, the universe had cooled sufficiently to form stable protons and neutrons. Then, protons and neutrons can fuse to give deuterium (H-2), isotope of hydrogen, nuclei. Then, deuterium nuclei fused to give stable He-4. After 3 minutes after the big bang, the universe is too cool for nuclear fusion to continue, so only light nuclei were formed.
After that, the gravity causes accumulation of hydrogen leading to star formation. This process leads to stellar nucleosynthesis, nuclear fusion in stars. In this process, heavier elements can be formed and only the first 26 elements are formed by fusion in"normal" stars with the most stable nucleus is Fe-56. The rest of the elements require more extreme condition to form, such as supernova. From those processes, we have the abundance of elements as shown below.
 |
The elements abundance in the solar system |
Firstly, we have a look about the structural of elemental solid class of
s and
p-block elements. The first class is metallic solid comprises of positive "cores" of metal atoms held together by surrounding "sea" of delocalised electrons or known as metallic bonding. The mobility of valence electrons in metallic structure leads to electrical conductivity. Secondly is molecular solid which is a solid composed of discrete molecules held together by intermolecular interactions and the examples are shown below.
 |
From left to right: P4, S8, I2 crystal structure, C60 |
The last class is covalent network solid which is a giant network of atoms linked by covalent bonds, such as diamond.
 |
Diamond crystal structure |
Hence, the rest of periodic table of
p-block elements are simple covalent molecules, such as N, O, group 17 an 18 elements.
Secondly, the periodic trends in atomic radius and there are different ways to measure it. Firstly is van der Waals radius which is the half the internuclear distance between non-bonded atoms that are in contact. Secondly is covalent radius which is measured as the half the internuclear distance for an X-X single covalent bond. Thirdly is ionic radius which is the internuclear distance between oppositely charged adjacent ions in an ionic lattice is the sum of their ionic radii. Lastly is the metallic radius which is half the internuclear distance between adjacent atoms in a metallic lattice. Those atomic radius definitions are shown below.
 |
Atomic radius definitions (upper line left to right): van der Waals radius, covalent radius
(lower line left to right): ionic radius, metallic radius |
The trends of atomic radius is generally decreasing across a period. This is caused by greater attraction of electrons by increasing nuclear charge as well increasing the number of electrons, but the electrons do not fill at higher energy, so there is no shielding effect. Besides that, the atomic radius increases as down the group because electrons enter higher shells, so it is shielded from nucleus by core electrons.
 |
Covalent radius from H to Cl (left) and ionic radius of group 1 and 2 (right) |
After that, we will see the trends when the elements loss its electrons or gain another electrons. To begin we start from the ionisation energy. Ionisation energy is the energy required to remove an electron from an atom in gas phase, so the equation is shown below for the first ionisation energy.
The trend of 1st ionisation energy across the periodic table is minima at group 1 and maxima at group 18, and increase across a period due to increasing nuclear charge. The increasing nuclear charge provides higher interaction with the outer electrons, so it requires energy to remove it. Besides that, it has dips at group 14 and group 16. Group 13 has lower ionisation energy than group 2 because the electrons in group 13 start to fill p-orbital which decrease the stability. Moreover, group 15 has higher energy than group 16 because group 16 electron configuration start to pair up and increase the repulsion. Meanwhile,
p electrons of group 15 are parallel spin. As down the group, the first ionisation energy decreases as the electron fills higher shells, so it is shielded by core electrons which makes easier to ionise. In group 13 it has less regular pattern as 3f transition occur between Ca and Ga, and this is caused by as Z increased by 10 but the 10
d-electrons do not shield very well, so electron at 4p of Ga is harder to remove.
 |
1st ionisation energy from H to Ar (left) and 1st ionisation energy of group 1, 2, and 13 (right) |
Besides ionisation energy, another atomic properties are electron affinity which is the energy given out when an electron is added to an atom in gas phase.
As additional note, the enthalpy change for this process is the electron attachment and the relationship is shown on the equation. Therefore, if the enthalpy is negative (exothermic), so EA is positive. The general trend is increase across period and there are dips at group 2 and group 15. The reason a dip at group 2 is due to full
s orbital, so an extra electron goes into p orbital of higher energy. Moreover, a dip at group 15 due to half filling of
p orbitals, so an extra electron has to pair. The trend as down the group is decreasing due to shielding by core electrons. However, low values for oxygen and fluorine is due to very small size of the atom. Hence, the added electron is repelled, leading to lower than expectation. Moreover, the second electron attachment enthalpy of oxygen is endothermic, so 2nd EA of oxygen is negative.
 |
1st electron attachment (EA1) of H to Cl (left) and 1st electron attachment of group 16 and 17 (right) |
Next properties is electronegativity (χ), which is the power of an atom in a molecule to attract electrons to itself (i.e. the electron withdrawing power of an atom) and there are 3 main scales of electronegativities:
- Pauling scale (χP) which is derived from bond dissociation energies, accounting for the contribution of bond polarity (more detail please check this link). A difference in electronegativity between 2 elements in a molecule leads to a polar bond.
- Alfred-Rochow scale (χAR) is related to the electrostatic force exerted by the nucleus on the valence electrons.
- Mulliken scale (χM) is the arithmetic mean of the first ionisation energy and the first electron affinity.
- Allen (spectroscopic) scale (χspec) is the average one electron ionisation energy of the valence shell s and p electrons.
The trend of electronegativity increases across period as the nuclear charge increasing and decreases down a group. Moreover, less regular pattern occur on 4th period and higher period due to insertion of
d and
f-block.
 |
The Allen electronegativity scale |
The value of electronegativity also can be used to predict the metals, non-metals, and metalloids elements.
In general, those classes has properties:
 |
χspec and metals, metalloids, non metals distribution |
Metals
- high electrical conductance which decreases with increasing temperature
- highly reflective
- strong, malleable, and ductile
- range of melting points
- low electronegativity (χspec < 1.9)
- valence electrons are held relatively weakly
- mobile (delocalised) electrons allow electrical conductivity
Metalloids
- semiconductors (intermediate electrical conductance, which increases with temperature)
- intermediate electronegativity (1.9 < χspec < 2.2)
- extended covalent networks - less electron delocalisation
Non-metals
- electrical insulators
- high electronegativity (χspec > 2.2)
- valence electrons are held relatively strongly
- electrons often shared in covalent bonds but are localised.
Hence, the distribution of those characters in periodic table of
s and
p elements are shown below.
Comments